What quantum neuroscience can learn from biophysics
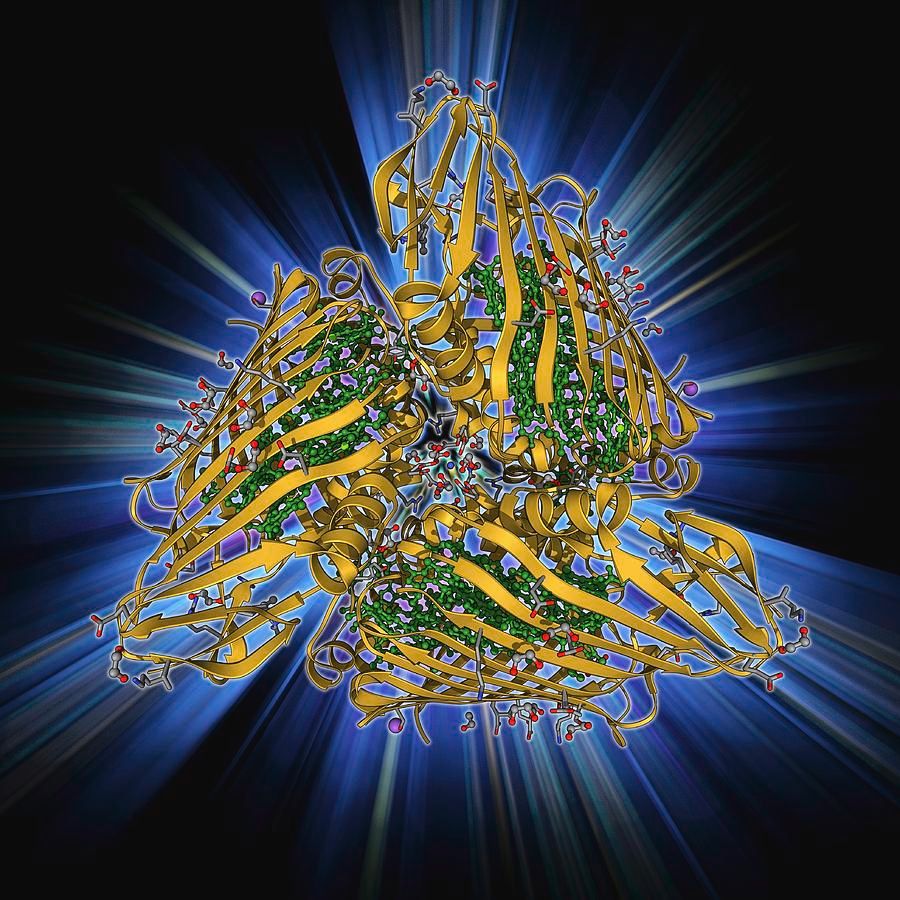
As the experimental evidence for quantum coherence in the photosynthetic apparatus increases, the quantum brain (and quantum mind) people will feel some sense of vindication. After all, they were the ones proposing quantum physics could persist in biological systems. Victory for the Hameroffs and Kauffmans of this world? Not quite.
The science weblogs and zines were buzzing with the headline. Mohan Sarovar and colleagues at the Department of Chemistry at Berkeley University have shown quantum entanglement in a photosynthetic protein (Sarovar et al., 2010). Or, well, they ran some simulations showing that entanglement was feasible in a given protein and partly robust to temperatures of 300 K. The attention was warranted – this is exciting stuff and everyone who cares about the biophysics of photosynthesis is anxiously awaiting for experimental verification of the model.
However, quantum biology findings also tend to lead to buzz about quantum neuroscience, and this is less warranted. For example, there is a proposal by biologist Stuart Kauffman, who argues free will stems from the brain equilibrating between a quantum and a classical state. One of his arguments is that quantum coherence is shown in photosynthesis and that it is therefore possible to find the same effect in the brain. The Sarovar research is likely to be added to the list of citations inspiring advocates of the quantum mind.
Of course, this is not wholly unreasonable. When Roger Penrose started writing his book on quantum gravity brain , he was critiqued by physicists and chemists for applying phenomena known from ultra-cold states of matter to systems above room temperature. For example, Tegmark threw calculations at Penrose showing thermal decoherence would destroy any quantum states in the living brain (Tegmark, 2000). If evidence shows coherence at room temperature, surely this argument is thrown off the table?
The answer is yes, but not completely. What Tegmark and others showed holds true, but with sufficiently advanced technology, you can stabilize the quantum coherence. As it turns out, photosynthetic machinery is such advanced technology. Done just right, biological organization can stabilize quantum coherence or even, as Sarovar and colleagues now suggest, quantum entanglement.
The "done just right" part is important. The Fenna-Matthews-Olson (FMO) protein complex used by Sarovar's team can be considered a model protein for investigating how photonic energy is transferred to a reaction center where it is turned into a chemical potential. But it forms a very specialized chemical milieu. The distance between chlorophyll molecules in the complex, the angles with which they face each other and the protein segments, the energy levels that they can occupy – all are carefully tuned parameters that allow the FMO to display quantum phenomena. The photosynthetic apparatus is a piece of high-tech equipment, as you would expect from an organism's energy core.
This should be taken into account by anyone advocating a quantum view of the mind. To display coherence at physiological temperatures, you need dedicated machinery. Without a plausible substrate for the quantum effect, there is no case. In the case of photosynthesis the FMO — of which the molecular and electronic structure is well known — is this substrate. Quantum mind theorists need to show their protein complexes or other structures of matter in which quantum states can persist.
However, even finding such a substrate is enought enough. Imagine that parts of the FMO complex would have been found to be coherent, but only parts that played no functional role. There would be a still be a substrate for quantum coherence in that case, but the coherence would not have contributed to the light-harvesting capability of the photosynthetic apparatus and it would therefore not have been sensible to speak about quantum biology. After all, the quantum level explanations would have no bearing on the biological behaviour of the molecules in question.
This point is especially important for quantum theories of mind, as these invoke quantum processes to explain grand questions such as free will, non-computational cognition or that holy grail: consciousness. Even if a substrate for quantum coherence would be found, quantum mind theorists would still need to connect that substrate to biological and cognitive effects.
Therefore, if quantum mind theorists are going to take anything from the findings on the FMO complex, it shouldn't be that "coherence is possible at 300 K". Rather, they should realize that for their own quantum biological processes, they need to find substrates, find an effective role for quantum coherence and then tie that role to a biological function. Only by taking the FMO research seriously as a model of what quantum biology should look like can quantum neuroscience escape from its current position as fringe science. I do not expect that to happen, but I'd love to be proven wrong.
References
Sarovar et al. (2010) Quantum entanglement in photosynthetic light-harvesting complexes. Nature Physics. DOI: 10.1038/nphys1652
Tegmark (2000) Importance of quantum decoherence in brain processes. Physical Review E 61: 4194–4206
Member discussion